Universidade Fernando Pessoa
Porto, Portugal
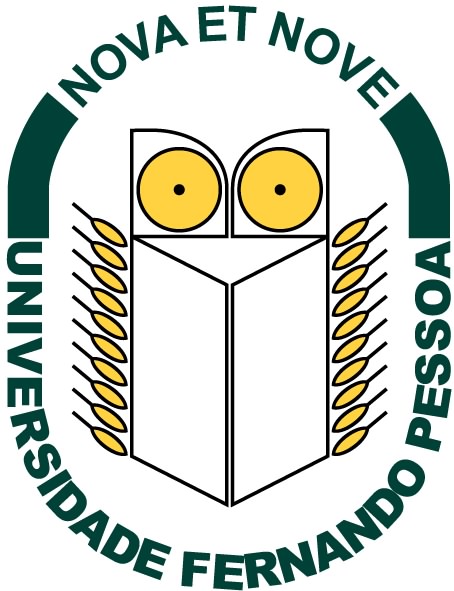
Salt Tectonics Short Course
6- SaltWelding
Contents:
6.1- Concept of Salt Welding
6.2- Types of Salt Welding
6.2.1- Primary Welds
6.2.2- Secondary Welds
6.2.3- Tertiary Welds
6.3- Recognition of Salt Welds
6.4- Salt Weld and Fault Weld
6.5- Translation Onlap Surfaces
6.1- Concept of Salt welding
A salt weld is a surface separating two stratal units formerly separately by salt and now in contact, as illustrated in fig. 183.
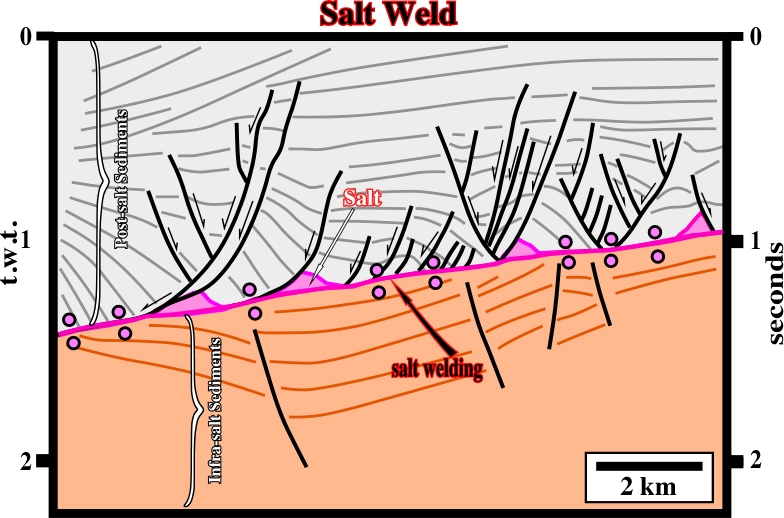
Fig. 183- On this line more than 80% of the salt was removed down-dip by flowage creating a discordantly welding between the supra-salt structures to sub-salt strata.
6.2- Types of Salt Welding
There are three types of salt welds:
(i) Primary Welds, (ii) Secondary Welds and (iii) Tertiary Welds
6.2.1- Primary Welds
Primary welds join strata originally separated by autochthonous salt (fig. 184). The welds are generally gently dipping. Joining regional dipping sub-salt strata with supra-salt sediments (overburden), which, locally, dip more steeply, creates a tectonic disharmony, which may look like an angular unconformity (fig. 185). Dip is locally enhanced by rotation due either to listric faulting or salt withdrawal below lapouts. Pitfalls produced by this process are: (i) Apparent downlaps (fig. 186 and 187) and (ii) Pseudo-turtle back structures (fig. 188 and 189). The associated disconformity is enhanced by rotation of the overburden creating apparent downlaps. The original onlaps are tilted, generally, landward as salt withdrawal.
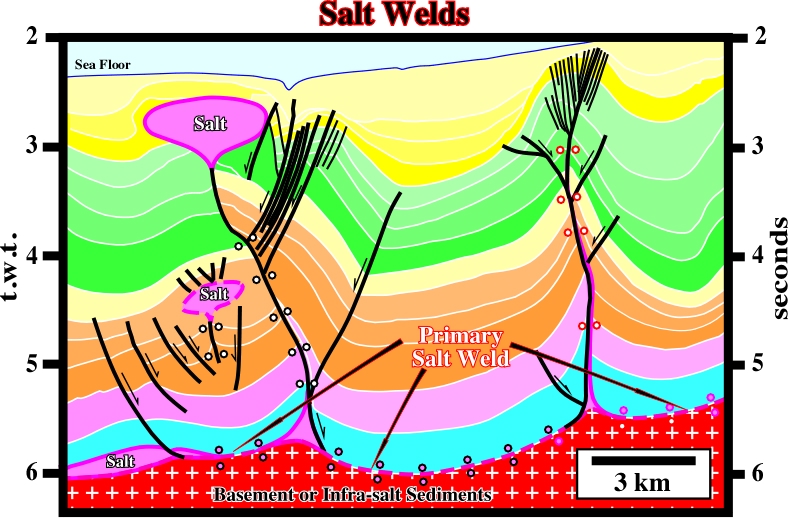
Fig. 184- On this seismic line, from the deepwater Angola, different types of salt welds can be recognized. Primary salt welds are those with a sub-horizontal geometry. They are associated with a removal of autochthonous salt, which can be perceived on the left end of the line.
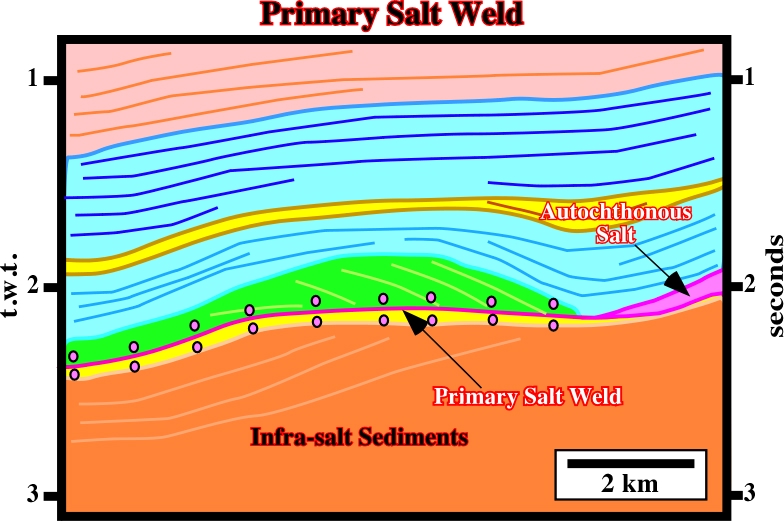
Fig. 185- The salt weld, created by the salt withdrawal of autochthonous salt, is highlighted by the small pink circles. On the right side of the line, autochthonous salt is seismically present. At the bottom of the overburden, the pristine onlap geometry of the seismic markers was deformed into apparent downlap reflection terminations.
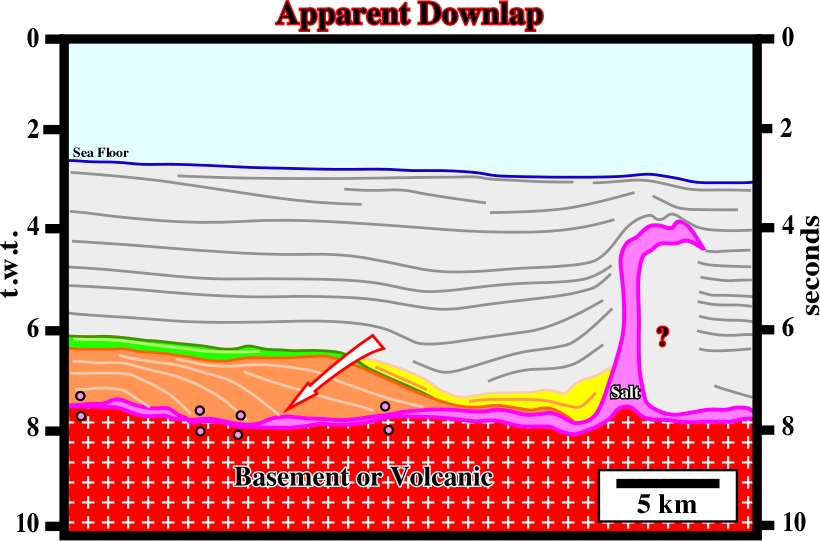
Fig. 186- The brown interval does not correspond to a progradational sedimentary package. The refection terminations do not define a downlap seismic surface. The seismic markers were deformed, and tilted eastward, due to salt flowage, which is also responsible for the allochthonous salt visible on the right side of the line. In the 80’s, the majority of the geologists working in the GOM interpreted the brown interval as the seaward progradation of the Cretaceous shelf break.
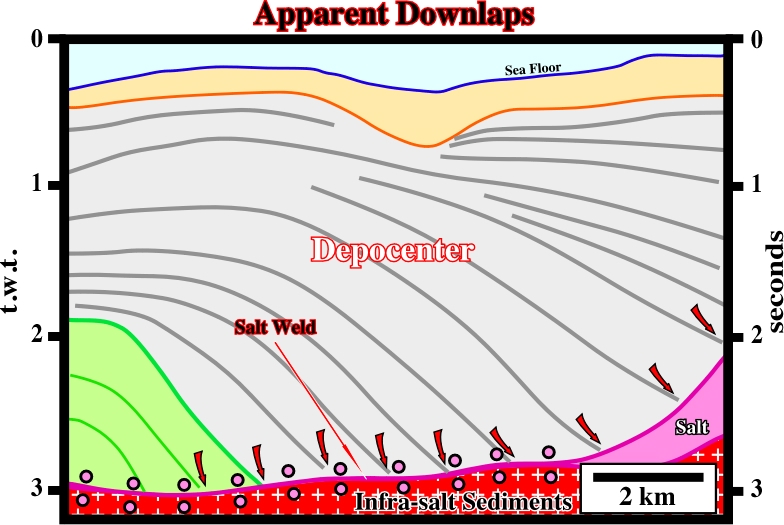
Fig. 187- It is quite simple to differentiate an apparent downlap surface from real one. The seismic interval between two consecutive progradations, roughly, a depositional system has a convergent geometry up dip and down-dip, with a maximum thickness between. Conversely, the interval between two consecutive apparent downlap progressively shows down-dip thickening, toward a growth fault plane or a salt weld.
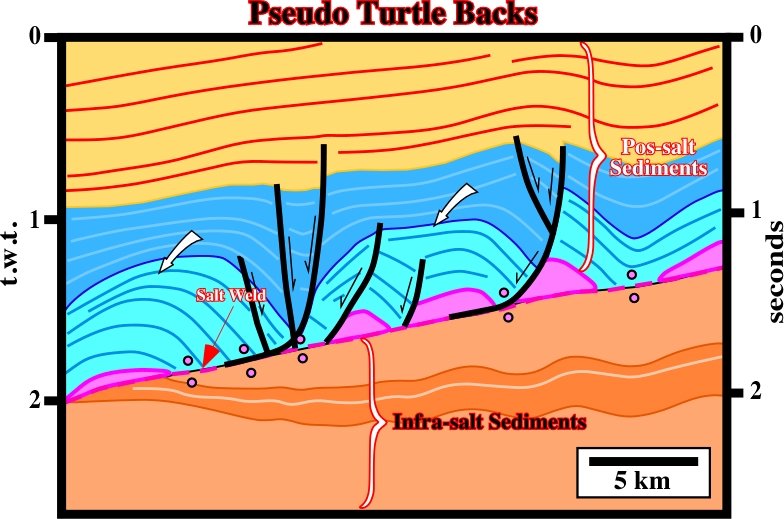
Fig. 188- The rotation of the overburden, above a primary salt weld induces antiform structures that should not be taken as turtle back structures. Indeed, it is easy to see that the sedimentary thickening of these structures is always toward fault looking seaward. Generally, there is not opposite thickening (seaward), as in a true turtle-back structure (convergence in stage 2 and divergence in stage 3).
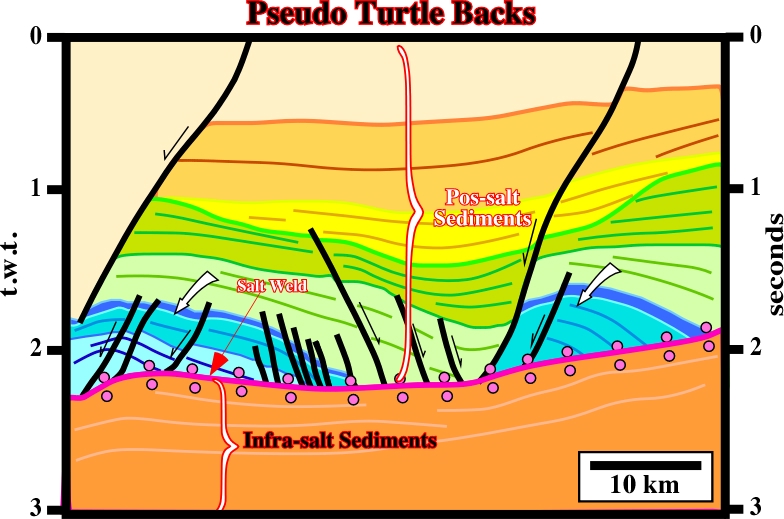
Fig. 189- On this seismic line from offshore Congo, the antiform structures are pseudo turtle-backs. They are associated with a salt flowage or with rafting (see later). Any significant tectonic inversion took place in their structural evolution.
6.2.2-Secondary Welds
Secondary salt welds join strata originally separated by steep-sided salt diapirs (walls, stocks, etc.), and are near vertical or are steeply dipping. The salt feeding a spreading bulb or allochthonous sheet causes the diapir stem to laterally shrink: (i) eventually thinning to negligible width or (ii) pinching off entirely (fig. 190), often by varying amounts of contraction.
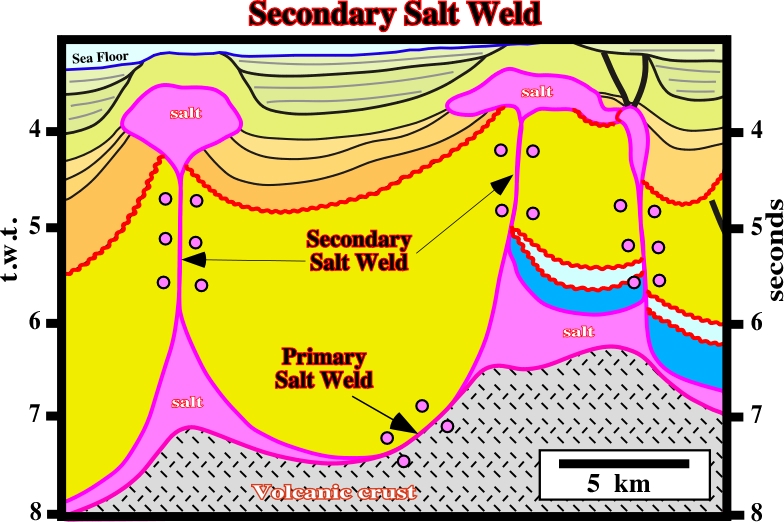
Fig. 190- Three bulbs seem to be connected with the autochthonous mother source layer by secondary welds. A more or less horizontal primary weld is also recognized.
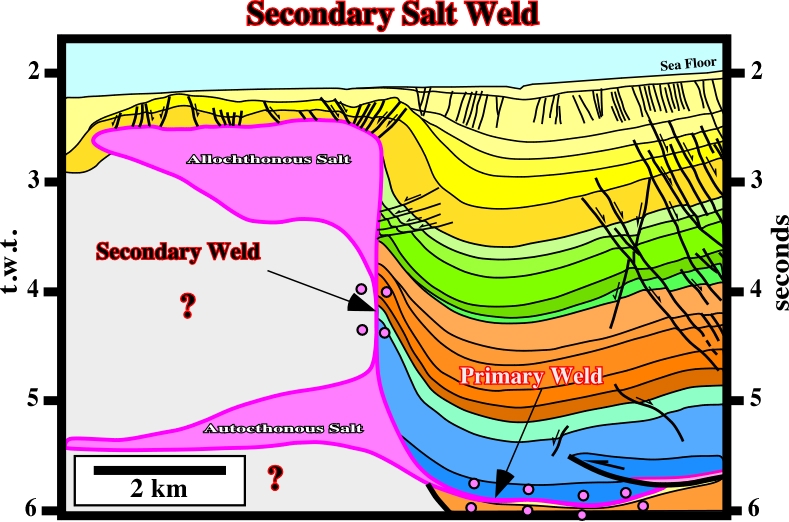
Fig.191- A secondary salt weld separates the allochthonous salt from the allochthonous layer.
The cover flanking each side of a diapir can then join discordantly together because diapiric growth is usually asymmetric. In such case, the salt weld resembles a growth-fault whether or not such a fault localized the formation of the diapir. This type of weld is often called fault weld.
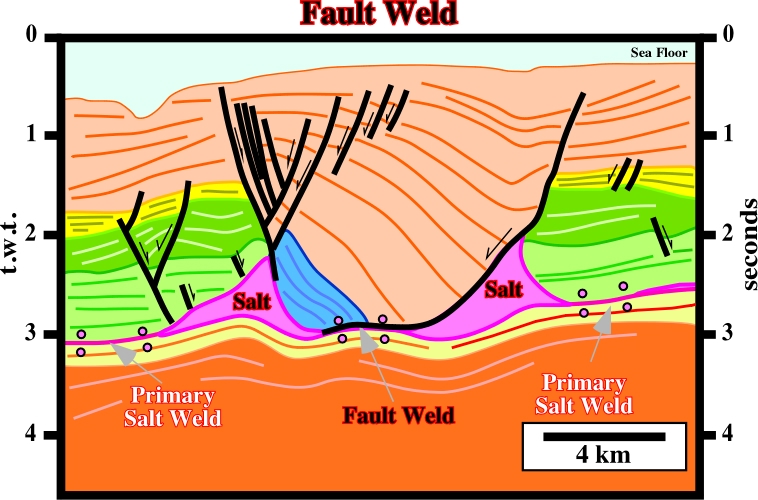
Fig. 192- Between two primary salt welds, along which no lateral displacement took place, there is a fault weld associated with the fault plane of a major growth-fault.
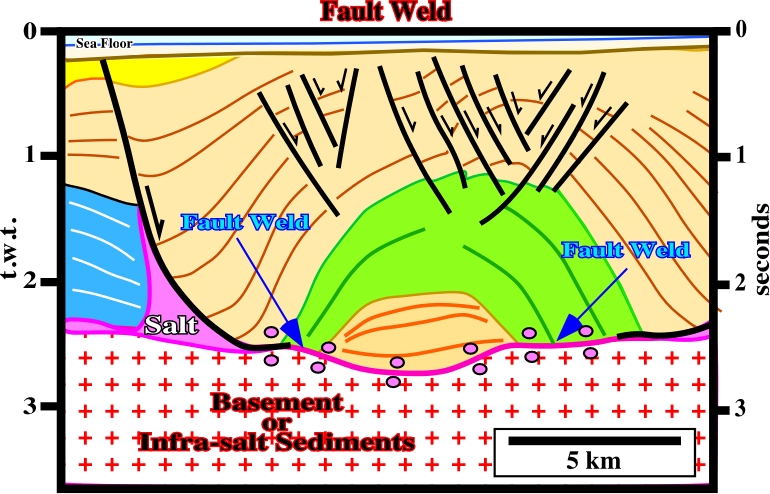
Fig. 193- The rotation of the chronostratigraphic reflectors within the downthrown faulted block strongly suggest a significant movement along the major fault plane, therefore along a fault weld. The fault plane roughly localize the eastern flank of the original salt diapir, which at present time is reduced to a salt roller.
6.2.3- Tertiary Welds
Tertiary salt welds (fig. 194 and 195) join strata originally separated by a first order or higher allochthonous salt sheet (canopies, tongues, nappes, sills, etc.).
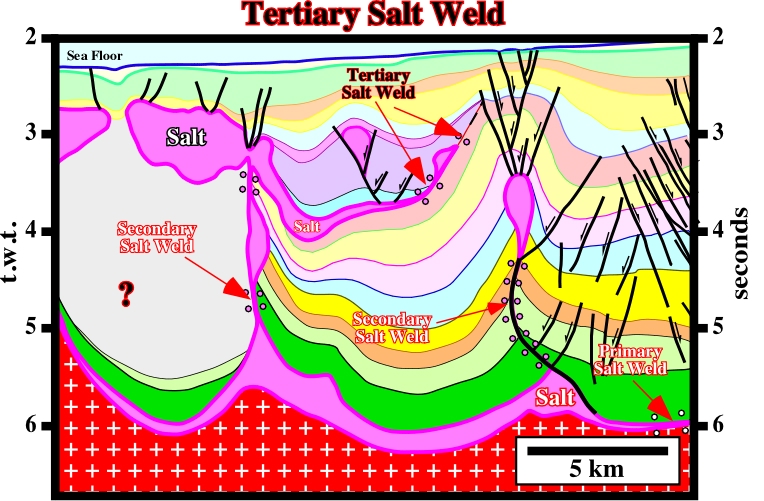
Fig. 194- The principal types of salt welds are recognized on this line from deepwater Angola. A tertiary salt weld is quite evident on the allochthonous sheet, in the upper part. The geometry of the two vertical secondary welds, recognized in the central part of the line, suggests the diapiric salt bodies were shortened. A primary salt weld is visible on the right bottom of the line.
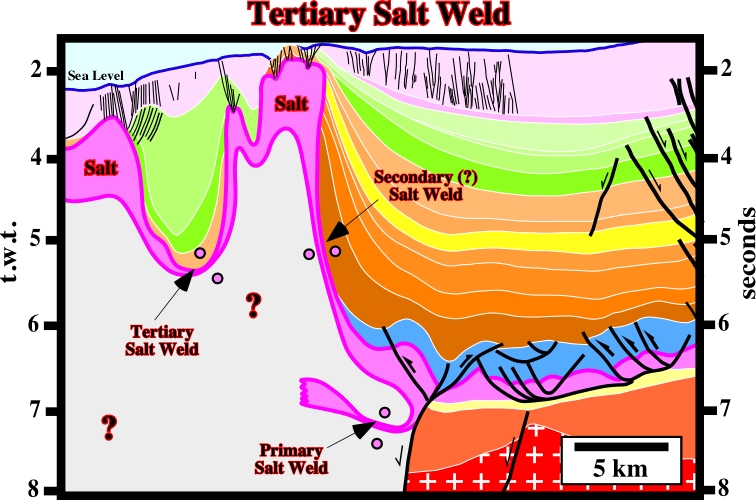
Fig. 195- At the bottom of a mini basin (or salt expulsion basin) created by allochthonous salt flowage a tertiary weld contrasts with the primary weld, at the bottom of the line, on the autochthonous salt.
Tertiary welds are generally shallow dipping. They are typically discontinuous and inserted at unpredictable stratigraphic and structural levels. They are particularly useful to mark the former position of allochthonous salt sheet. Tertiary welds are generally overlain by highly extended cover and may be fronted by shortened strata, what leads to complex mixture of structural features on each side of the salt weld.
6.3- Recognition of Salt Welds
The recognition of a salt weld, as schematized on fig. 196, is quite important to understand the geology of salt basins and the associated petroleum systems.
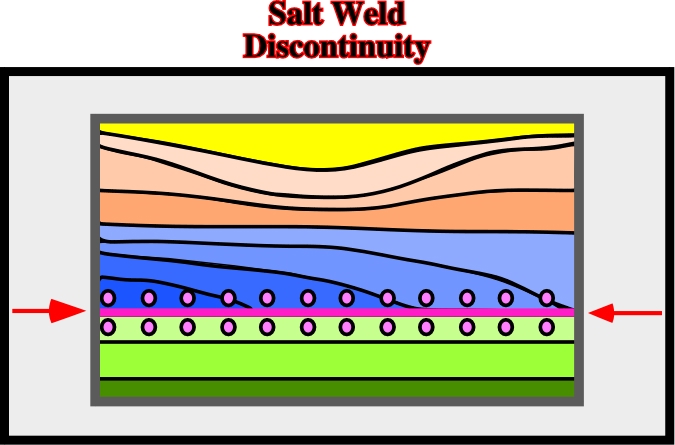
Fig. 196- Salt welds allow recognizing deep, thin and vanished salt. When ignored, there is a misinterpretation of the structural history and sequence stratigraphy. When they are combined with extension, they become complex and overestimations of salt reduction are frequent (fig. 197).
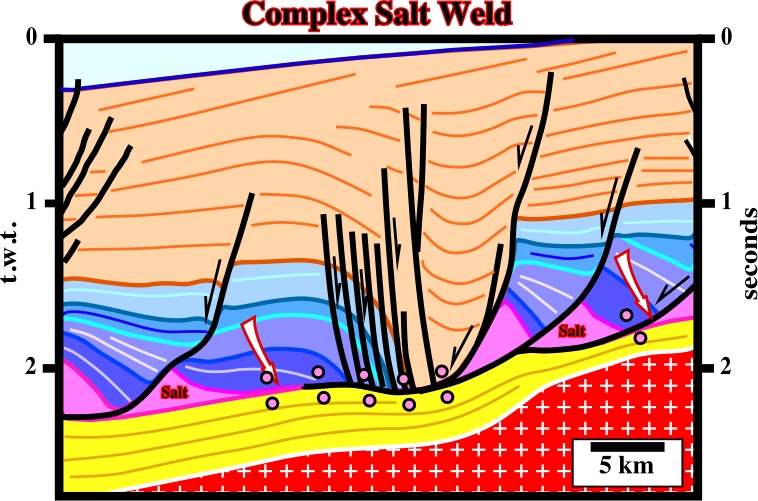
Fig. 197- When welding (secondary or fault weld) is combined with extension, as illustrated on this line, one should not overestimate the salt reduction (see later).
6.4- Salt Weld and Fault Weld
A salt weld is a surface joining rock volumes formerly separated by salt as illustrated in fig 198.
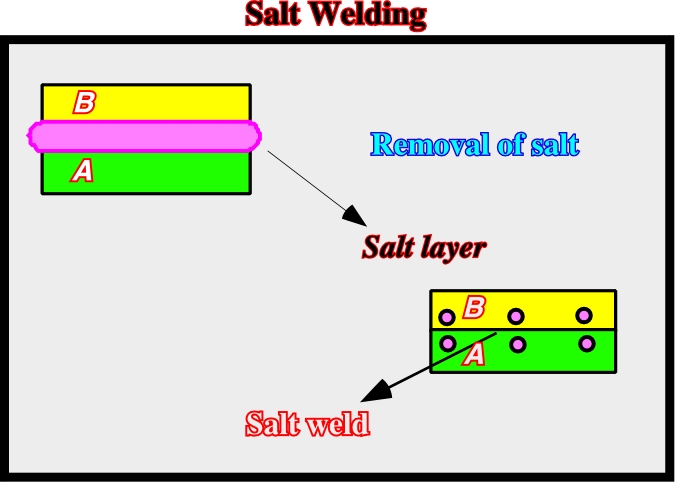
Fig. 198- Salt welding does not imply relative movement between the overburden and the sub-salt strata, but a total salt removal, which could not be the case on the seismic lines. At the seismic standpoint, a salt thickness under seismic resolution is considered a weld (see fig. 199).
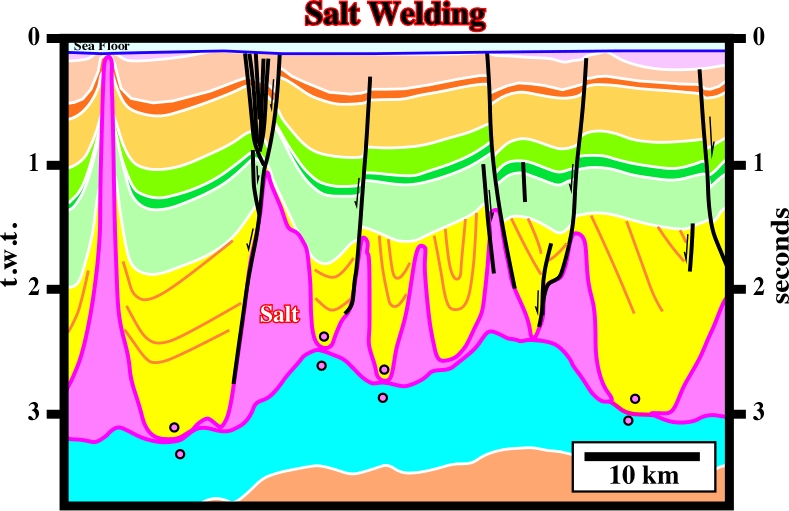
Fig. 199- In spite of the geometry of the synkinematic layer (in yellow), the salt welds recognized on this line do not involve a significant lateral displacement. Wells found a thin salt interval (under seismic resolution) on the welds.
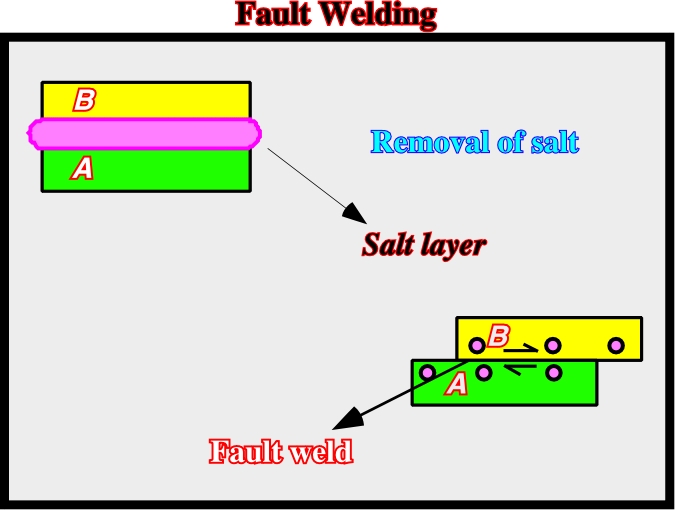
Fig. 200- As previously (fig. 198), in a fault weld, which implies a relative lateral displacement between the overburden and the sub-salt strata, the salt removal is related to seismic resolution (fig. 201).
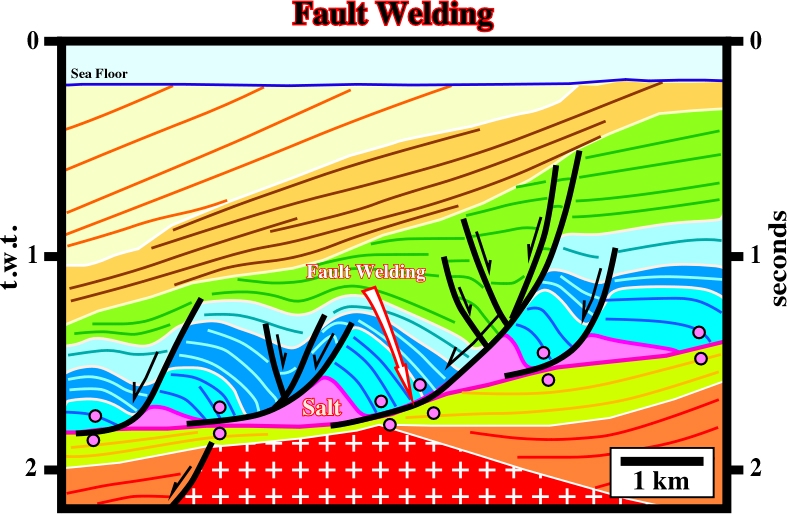
Fig. 201- In certain areas of the Kwanza and Congo basins, an extensional tectonic regime is associated with halokinesis. Therefore, salt tectonics becomes dominant. Indeed, not all structures can be explained just by halokinesis. On this line one can recognize: (i) secondary welds, (ii) growth faults, (iii) rollovers and (iv) compensation grabens, (v) fault welds, etc. (see next chapter).
6.5- Translation Onlap Surfaces
Translation of the overburden across a stepped salt detachment (thin salt) bends the post-salt sediments and can create apparent translation downlap surfaces (gliding onlaps) in the synkinematic layers (fig. 202 to 205).
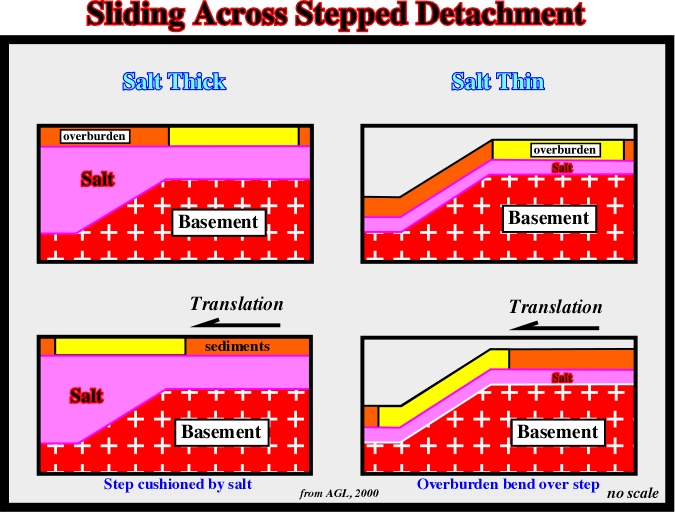
Fig. 202- When the salt is thick, a step at the bottom of the salt is often cushioned by the salt itself and thereof the overburden is translated basinward without deformation. If the salt is thin, a prekinematic overburden layer may bend over the step, since the salt thickness cannot cushion it.
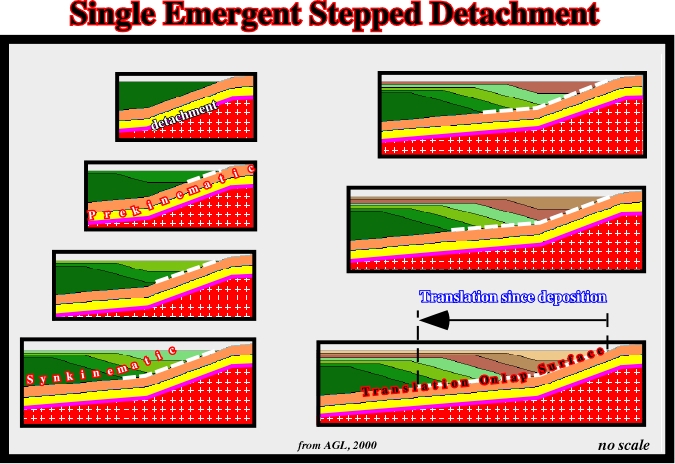
Fig. 203- Due to the translation along the detachment surface, the pristine depositional onlaps of the synkinematic overburden layer progressively build up an apparent downlap surface. The distance between the first apparent downlap and the step gives the total translation.
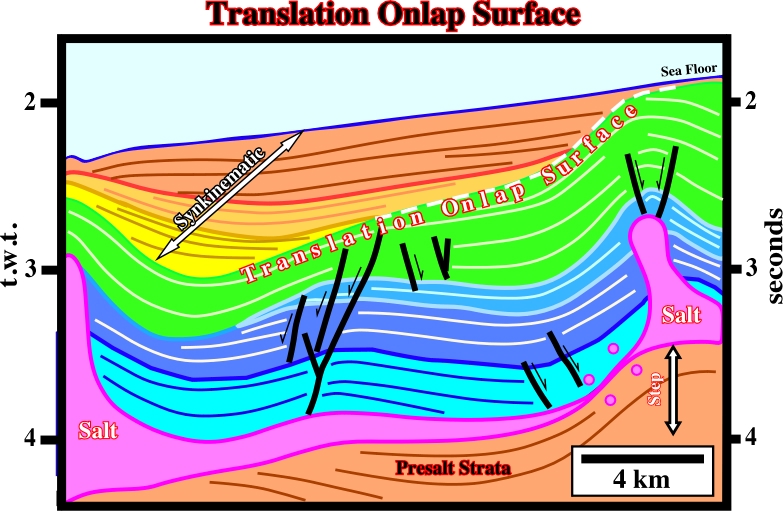
Fig. 204- The apparent downlap surface created by translation of the overburden across a single emergent step, at the bottom of the salt, recognized on this line, is quite characteristic. In spite of the fact that it is directly associated with a salt step, its genesis is quite different than that of a salt weld (see fig. 205).
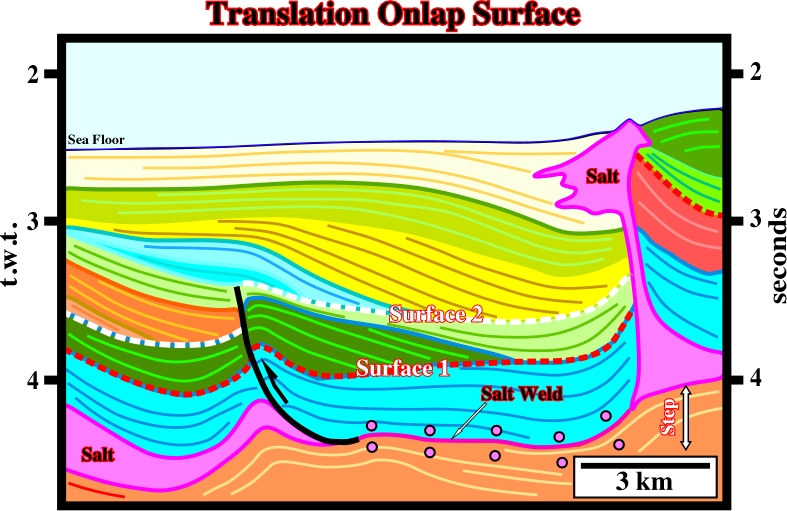
Fig. 205- In particular cases, depending on tectonic evolution and salt tectonics, several translation onlap surfaces (or apparent downlap surfaces) can be recognized in the synkinematic overburden (synchronous of the basinward translation). Such a surfaces are not associated with a salt withdrawal or salt flowage, therefore they are not salt or fault welds. Complex cases with two salt steps and diapirs will be described in a later chapter.
Summing up, the key points of overburden translation over a single buried step can be summarized as follows:
a) If the sedimentation rate is insufficient to cover the bathymetric escarpment, each unit will onlap above the step.
b) Onlaps are translated basinward after deposition, producing a landward-dipping package of onlapping strata (apparent downlap surface).
c) The distance from a given onlap to the step records the amount of translation since the deposition of that unit.
d) As older depocenters are translated basinward, younger depocenters form at the step. Continued translation produces a shingled series of landward-dipping stratal units, bounded by two growth axial surfaces.
c) The distance between the step and the intersection of a horizon with the landward growth axial surface records the translation since the deposition of that horizon.
d) For a given translation rate, faster sedimentation produces steeper growth axial surfaces.
On this subject, it is interesting to note that the salt steps recognized in the offshore Angola (previous examples) seem to correspond to the fracture zones (see later) separating the different geological provinces of the South Atlantic margins. However, the one illustrated on the seismic line below (fig. 206), apparently does show any evident translation onlap surface.
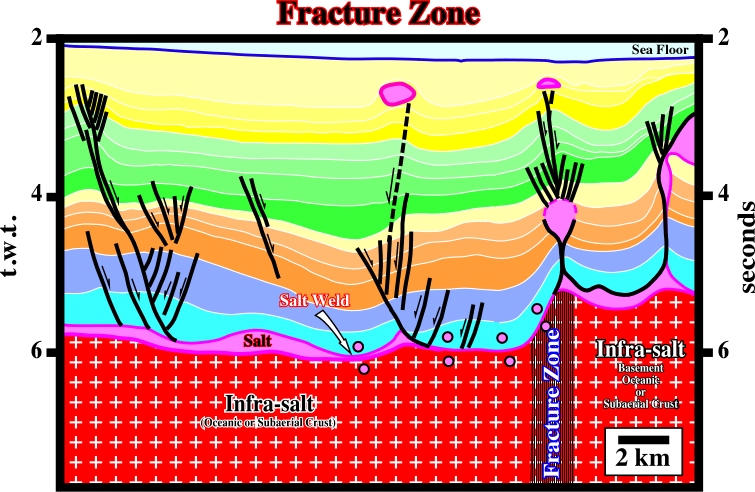
Fig. 206- Taking into account the orientation of this line, which is more or less perpendicular to a potential basinward translation of the overburden, it is possible that a translation onlap surface is masked. Another possibility is that basinward translation did not occur in this area.
to continue press
next

Send E-mail to carloscramez@gmail.com with questions or comments about these notes.
Copyright © 2006 CCramez
Last modification:
August, 2014.